The quest for achieving practical nuclear fusion has driven the exploration of various types of fusion, each offering a distinct methodology and holding its own promise for future energy production.
At its core, nuclear fusion involves the merging of two light atomic nuclei into a heavier nucleus, releasing an enormous amount of energy.
This process contrasts with nuclear fission, where heavy atomic nuclei split into lighter ones.
Fusion promises a virtually limitless supply of energy with minimal environmental impact, making it a key player in the future of energy production.
Magnetic Confinement Fusion (MCF)
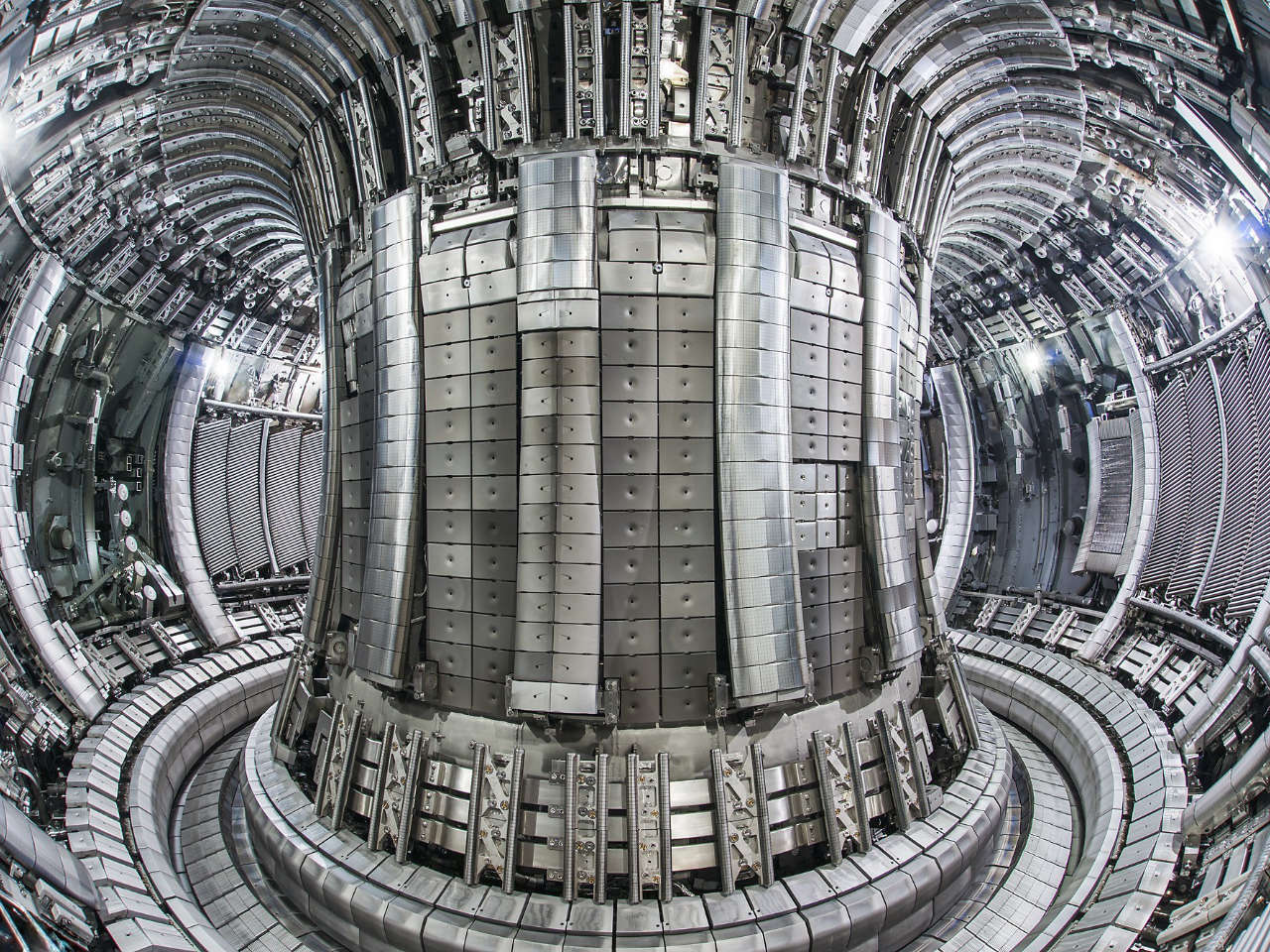
Tokamak Reactors: The best known type, using powerful magnetic fields to contain hot plasma in a chamber.
Stellarators or Helical Reactor: Similar to tokamaks but with a twisted magnetic field configuration, potentially offering better stability.
These reactors hold immense promise as a future clean energy source.
Here’s a breakdown of how it works:
Fuel and Heating
MCF uses a fuel of isotopes of hydrogen – deuterium and tritium.
These are heated to extremely high temperatures, much hotter than the sun’s core, to form a hot, charged gas called plasma.
Magnetic Confinement
This superheated plasma can’t be contained by any traditional material because of its high temperature, (over 100 million Celcious).
Instead, MCF uses powerful magnetic fields to confine the plasma.
Imagine a giant, invisible donut – that’s the typical basic shape the magnetic field creates.
The doughnut-shaped field is called a torus.
The strong magnetic forces accelerate the charged particles in the plasma around the tooidal shaped field, preventing the plasma from touching the walls of the reactor, which would quench the plasma and stop the fusion reaction.
Fusion Reaction
Within the confined plasma, the nuclei of the deuterium and tritium atoms fuse together, releasing a tremendous amount of energy in the form of heat.
This is the same process that powers the sun and other stars.
The principle of achieving fusion by MCF has been proven with several large scale, short term experiments and the current challenge is to design and build a commercially viable fusion facility with an acceptable system life time.
Inertial Confinement Fusion (ICF)
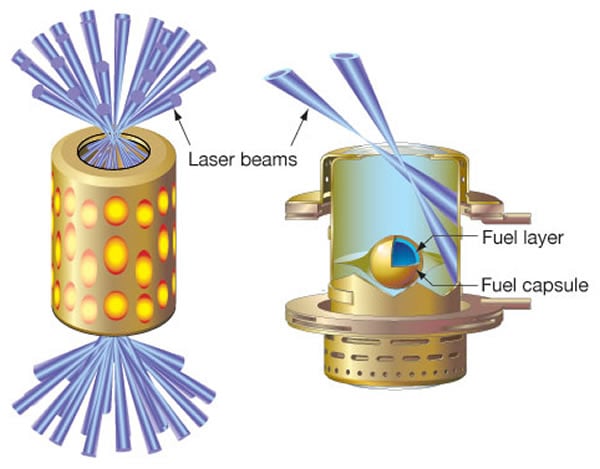
Inertial Confinement Fusion (ICF) is another approach to achieving fusion on Earth, but instead of using magnetic fields, this type of nuclear fusion relies on extreme pressure and heat to create the necessary conditions.
Here’s a simplified breakdown:
Fuel and Target
Similar to MCF, ICF uses hydrogen isotopes (deuterium and tritium) as fuel.
These are packed into a tiny capsule.
High-Powered Lasers (or Ion Beams)
Powerful lasers (or sometimes ion beams) are fired at the capsule from all directions.
Compression and Ignition
The intense laser blasts rapidly compress and heat the fuel capsule, creating an incredibly hot and dense environment.
This triggers fusion reactions within the fuel, releasing energy.
Imagine squeezing a tiny balloon filled with fuel so hard and fast that it ignites.
The Race Against Time
Because the fuel isn’t confined by a magnetic field, the entire process needs to happen very quickly, in billionths of a second.
It’s a race against time to achieve fusion before the compressed fuel cools and falls apart.
ICF is a promising avenue for fusion energy.
Researchers are actively working on improving laser technology and designing more efficient ways to capture the released energy.
Magnetized Target Fusion (MTF)

Unlike MCF which relies on expensive superconducting magnets, MTF uses simpler and cheaper electromagnets for the initial confinement.
MTF hopes to achieve higher fuel densities compared to standard MCF, potentially leading to more efficient fusion reactions.
Here’s how it works:
Combines Concepts
MTF borrows elements from both MCF and ICF.
It utilizes magnetic fields for some plasma confinement, like MCF, but also relies on rapid compression for ignition, similar to ICF.
Fuel and Target
Similar to MCF, MTF starts with a preheated and magnetized plasma, often within a chamber. As with ICF, MTF uses hydrogen isotopes (deuterium-tritium) encased in a target which is usually in liquid form and injected into the chamber.
Magnetic Field and Compression
The target is placed within a chamber with an electromagnetic field.
This magnetic field confines the plasma to a certain extent, but not as strongly as in MCF.
Then, powerful drivers, like pistons or jets of liquid metal, rapidly compress the target.
Fusion Ignition
Fusion reactions release a burst of energy, which can be used to heat a surrounding material and eventually generate electricity
Since MTF operates in pulses, efficiently repeating the fuel injection, compression, and energy extraction cycle is crucial for a viable power source.
More research is needed to optimize the compression process and achieve sustained fusion reactions.
Cold Fusion
Cold fusion is a hypothetical process where atomic nuclei fuse at or near room temperature, in contrast to the incredibly high temperatures (millions of degrees) required for traditional fusion reactions (“hot fusion”). It has been a controversial topic since 1989 when reports of successful cold fusion experiments surfaced. However, there is no conclusive evidence to support its existence.
Advanced Fusion Concepts
Advanced fusion concepts refer to approaches beyond the main types of nuclear fusion mentioned earlier. Two intriguing advanced fusion concepts are Aneutronic Fusion and Fusion-Fission Hybrids.
Aneutronic Fusion
Aneutronic Fusion is a type of nuclear fusion where the goal is to minimize the production of neutrons. Unlike standard fusion reactions, which release a significant amount of their energy as neutrons, aneutronic fusion aims to capture energy from charged particles instead. This approach is more efficient reducing radioactive waste, simplify the reactor design and create electrical energy directly. The challenges include achieving the higher temperature required for this method and this technique will release less energy compared to the D-T reaction.
Fusion-Fission Hybrids
Fusion-Fission Hybrids combines aspects of both fusion and fission reactors into a single system. Fusion reactors can provide a source of neutrons, which can be used to “burn” fissile material in a subcritical fission reactor.
Overall, Aneutronic Fusion and Fusion-Fission Hybrids are innovative approaches that aim to address some of the challenges associated with traditional fusion reactors. However, both concepts are in the early stages of development and require significant research and engineering advancements before they become a reality.
Precision Ceramics and Fusion
For more information on the ceramics used in fusion, please visit our fusion page.
The extreme environments in the multiple fusion systems being developed mean that engineering ceramics will be required across the fusion core systems and the peripherals.
Even higher performing materials will need to be developed to survive the most challenging applications.
If you have any questions regarding which ceramic material might be best for your application, please don’t hesitate to contact us.
Related Topics
Ceramics for Fusion
At Precision Ceramics, our expertise in working with ceramics for Fusion reactors spans many decades, specializing in supplying a wide range of ceramics for use in fusion environments.
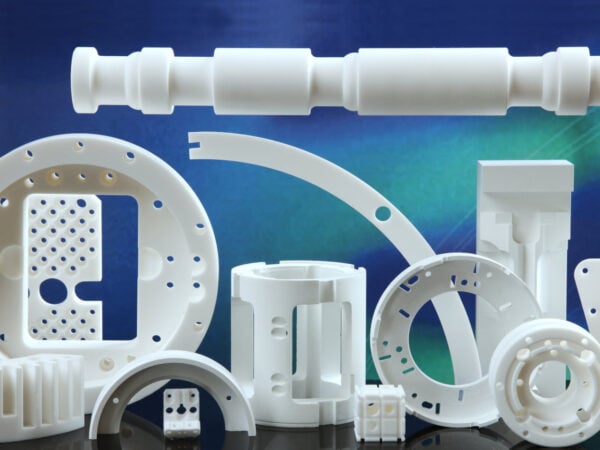
Materials
Precision Ceramics offers a wide range of advanced ceramic materials. We can help with the engineering tolerances, design for manufacture, selection of the materials and application environment questions.